In vivo verification of regional hyperthermia in the liver
Article information
Abstract
Purpose
We performed invasive thermometry to verify the elevation of local temperature in the liver during hyperthermia.
Materials and Methods
Three 40-kg pigs were used for the experiments. Under general anesthesia with ultrasonography guidance, two glass fiber-optic sensors were placed in the liver, and one was placed in the peritoneal cavity in front of the liver. Another sensor was placed on the skin surface to assess superficial cooling. Six sessions of hyperthermia were delivered using the Celsius TCS electro-hyperthermia system. The energy delivered was increased from 240 kJ to 507 kJ during the 60-minute sessions. The inter-session cooling periods were at least 30 minutes. The temperature was recorded every 5 minutes by the four sensors during hyperthermia, and the increased temperatures recorded during the consecutive sessions were analyzed.
Results
As the animals were anesthetized, the baseline temperature at the start of each session decreased by 1.3℃ to 2.8℃ (median, 2.1℃). The mean increases in temperature measured by the intrahepatic sensors were 2.42℃ (95% confidence interval [CI], 1.70-3.13) and 2.67℃ (95% CI, 2.05-3.28) during the fifth and sixth sessions, respectively. The corresponding values for the intraperitoneal sensor were 2.10℃ (95% CI, 0.71-3.49) and 2.87℃ (1.13-4.43), respectively. Conversely, the skin temperature was not increased but rather decreased according to application of the cooling system.
Conclusion
We observed mean 2.67℃ and 2.87℃ increases in temperature at the liver and peritoneal cavity, respectively, during hyperthermia. In vivo real-time thermometry is useful for directly measuring internal temperature during hyperthermia.
Introduction
Hyperthermia is known to be an effective radiosensitizer of hypoxic or S-phase cells, which are relatively resistant to radiotherapy (RT) [1,2,3]. Based on this rationale, several clinical studies have demonstrated the effectiveness of hyperthermia in combination with RT [4,5,6,7]. RT has been implemented as an effective local treatment modality for hepatocellular carcinoma (HCC) [8,9,10,11,12], and patients with HCC may benefit from combined treatment of RT and hyperthermia [13,14].
To achieve benefit from hyperthermia, temperatures in the target volume range of 40℃-43℃ and the capability of measuring the temperatures at the described locations are technical requirements for quality assurance [15,16]. Several methods have been introduced for temperature measurements [16,17,18], and the application of invasive thermometry has been particularly controversial because of the possibility of severe complications [19,20]. For pelvic tumors, such as rectal, cervical, and prostate cancer, intraluminal thermometry is a potential substitute for invasive thermometry [18,19]. In addition, magnetic resonance (MR) thermometry has been investigated as a non-invasive method [21,22].
However, there is an indispensable need for invasive thermometry in some selected cases [20]. Because the liver is a mobile and well-vascularized organ, achievement of temperature increase may be complicated. With the launch of prospective clinical studies using hyperthermia in combination with RT for primary or metastatic liver tumors, there is need to verify the local increases in temperature in the liver during hyperthermia. In addition, a newer type of hyperthermia delivery system that uses electromagnetic waves for regional heating needs to be verified before use in trials. To evaluate these issues, we performed invasive thermometry in pigs, which have similar thickness to humans.
Materials and Methods
We used three 40-kg pigs for our experiments. General anesthesia was induced by intramuscular injection of ketamine (20 mg/kg) plus xylazine (2 mg/kg) and was maintained by isoflurane vaporized at 2%. The muscle relaxant vecuronium (0.1 mg/kg) was administered intravenously to facilitate the long-term maintenance of anesthesia. The induction and maintenance of general anesthesia was conducted by an experienced veterinarian (SBH) and laboratory animal technicians (JHP). This study was reviewed and approved by the Institutional Animal Care and Use Committee (IACUC No. 20131226005) of Samsung Biomedical Research Institute (SBRI). SBRI is an Association for Assessment and Accreditation of Laboratory Animal Care International (AAALAC International) accredited facility and abide by the Institute of Laboratory Animal Resources (ILAR) guide.
Four glass fiber-optic sensors (Celsius TempSens) were used for measuring temperature. Under ultrasonographic guidance, two sensors were placed in the liver (intrahepatic #1 and #2), and one was placed in the peritoneal cavity in front of the liver by an experienced interventional radiologist (YSK). Another sensor was placed on the skin surface to assess superficial cooling. We placed intrahepatic #1 sensor, as well as the intraperitoneal and skin sensors, on central axis of the corresponding electrode (Fig. 1A).
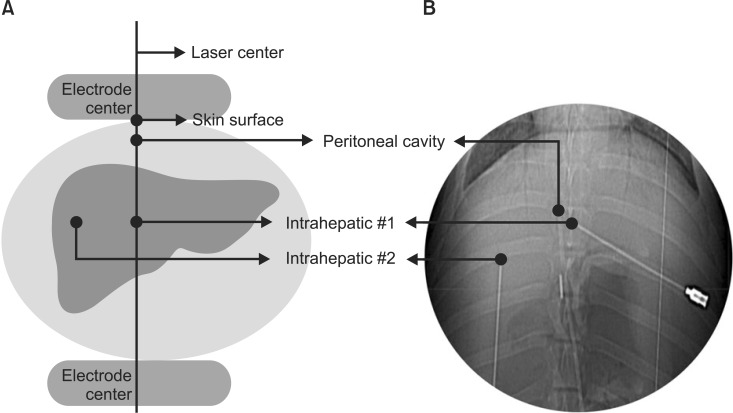
Locations of the fiber-optic sensors (A) were verified by computed tomography scan (B) after completion of the final session.
We used the Celsius TCS electro-hyperthermia system (Celsius42+ GmbH Company, Cologne, Germany) for hyperthermia. Hyperthermia was delivered according to the protocol for liver treatment offered by the manufacturer, which was also applied to our prospective clinical studies for liver tumors. As summarized in Table 1, it was composed of six sessions and the energy delivered was increased from 240 kJ to 507 kJ during the 60-minute sessions. The 1st and 2nd sessions were supposed to be delivered before the start of RT to facilitate patients' adaption for hyperthermia. Then, the 3rd session was scheduled to be delivered immediately after the 1st fraction of RT. While each session has interval for 2-3 days between each other, the experiment was performed consecutively with at least 30 minutes of the inter-session cooling periods because practical limitation of large animal experiment.
The diameter of the electrodes was 25 cm to fully cover the liver and all sensors, and then the sensors were located within the range of the electrodes. The temperatures at the four sensors were recorded every 5 minutes during hyperthermia and every 10 minutes during the intersession breaks. After completion of the final session, an abdominal computed-tomography scan was acquired to verify the locations of the sensors (Fig. 1B).
The primary endpoint of this study was the temperature change after the consecutive sessions. The temperature change was calculated as the difference between the temperatures before and after each session. Data from the experiments were summarized as mean ± standard error of mean, and the Wilcoxon signed-rank test was used to determine the statistical significance of the temperature changes. The descriptive analysis and graphing of the experiment results were conducted using GraphPad Prim 6.0 software (GraphPad Software Inc., La Jolla, CA, USA). SAS software (SAS 9.1.3; SAS Institute Inc., Carry, NC, USA) was used for the statistical analysis.
Results
A representative figure showing the recorded temperatures is shown in Fig. 2. The skin was kept at a low temperature during hyperthermia through the application of the cooling system. As the pigs were anesthetized, the baseline temperature at the starting point of each session decreased (Table 2 and Fig. 3). Therefore, the intrahepatic and intraperitoneal temperatures were not appreciably elevated during the first two sessions. As more sessions followed with intensified energy delivery, the increases in temperatures appeared to be more prominent (Fig. 3). The mean increases in intrahepatic temperatures were 2.42℃ (95% confidence interval [CI], 1.70-3.13) and 2.67℃ (95% CI, 2.05-3.28) during the fifth and sixth sessions, respectively. The corresponding values for the intraperitoneal sensor were 2.10℃ (95% CI, 0.71-3.49) and 2.87℃ (95% CI, 1.13-4.43), respectively.

Temperature recordings of a representative experiment. While the baseline temperature was decreased due to deep anesthesia of the pig, the intrahepatic and intraperitoneal temperatures were effectively increased during regional hyperthermia. The room temperatures during the experiment ranged from 23.6℃ to 25.5℃.
Discussion and Conclusion
In this study, we observed a mean 2.67℃ increase in intrahepatic temperature during the sixth session of regional hyperthermia. While the normal body temperature of a pig is known to be about 39℃, it was found to be about 36℃ in our experiments, as shown in Fig. 2. The body temperature of these pigs could have been affected by the room temperature during the experiments, which ranged from 23.6℃ to 25.5℃, as well as by the effects of general anesthesia accompanied by muscle relaxant. As shown in Fig. 3, the baseline temperature at the starting point of each session decreased by up to 2.1℃. Although the temperature increase achieved in our study is not likely to be sufficient to achieve therapeutic advantage [16], it could be complemented by the maintenance of baseline body temperature under conditions without anesthesia.
Because the liver is a well-vascularized organ, perfusion-mediated tissue cooling is a potential contributing factor for local temperature during hyperthermia [23]. According to a study by Nagata et al. [24], most liver tumors could not be heated to more than 42℃. Even though the thermometry results for liver tumors were not satisfactory, the treatment results were relatively favorable. The study authors performed invasive thermometry by placing a thermocouple in a catheter that was inserted into the liver tumor. However, the use of invasive thermometry was limited by bleeding tendency with poor liver function, tumor location, and presence of massive ascites. Serious sequelae included intraperitoneal hematoma and pneumothorax.
Similarly, Seong et al. [13] reported the results of the combined treatment of RT and hyperthermia for locally advanced HCC. Despite limitations, such as bleeding tendency, catheter obstruction due to blood clots, or misplacement of the catheter, successful thermometry was performed in 16 (19.0%) of 84 patients. The maximum, minimum, and average temperatures were 41.9℃ ± 1.3℃, 39.9℃ ± 1.0℃, and 40.8 ± 0.9℃ (mean ± standard deviation), respectively. Although the patients had advanced disease with tumors larger than 5 cm in diameter and the RT dose (30.6 Gy in 3.5 weeks) was relatively low in terms of the dose-response relationship [25], the response rate was 40.3%, including two patients with complete response.
In contrast to pelvic tumors, such as rectal, cervical, prostate, bladder, and anal cancer, where endoluminal temperature measurement is possible, the liver cannot feasibly be assessed with this method. Therefore, invasive thermometry is the only method that can precisely measure intrahepatic temperature. However, clinical application of invasive thermometry is practically limited, as previously described [13,24]. MR thermometry is a potential noninvasive alternative [21,22], although it is limited by the constant movement of the liver due to respiration [26]. The increased use of MR could cause difficulty in terms of facility requirements and cost. Because quality assurance is essential for hyperthermia delivery systems [16], studying in vivo real-time thermometry using large animals is worthwhile in order to directly measure internal temperatures during hyperthermia so as to avoid the potential risks of severe sequelae in patients. Furthermore, biochemical and histopathological changes after hyperthermia in liver can be assessed using animal experiments [27], while we did not conducted subsequent experiments. We observed a certain degree of temperature increase in the livers of the pigs during hyperthermia, although this was likely influenced by the room temperature and the state of deep anesthesia with the use of muscle relaxant. The thermometry results seem to be unsatisfactory, as reported in previous studies [13,24], but are not necessarily related to inferior outcomes. This question of efficacy may be answered by our currently ongoing clinical studies using hyperthermia in combination with RT for treatment of primary or metastatic liver tumors (NCT01911000 and NCT01963117).
There were several limitations of this study. First, decreased baseline temperature could be influenced by general anesthesia. Second, we assessed normal liver instead of tumor tissue. Temperature monitoring using tumor model should be considered in future. Third, we did not examine the histopathologic changes including fat necrosis, which could be induced by hyperthermia.
In conclusion, we observed mean 2.67℃ and 2.87℃ increases in temperatures at the liver and peritoneal cavity, respectively, during regional hyperthermia. Uncertainty as to whether the observed elevations in temperature are associated with improved clinical outcomes will need to be answered in future studies.
Acknowledgments
This study was supported by Samsung Medical Center grant (No. GFO1130081).
Notes
The hyperthermia system was rent from Celsius42+ GmbH Company for prospective clinical studies using hyperthermia in combination with radiotherapy for primary or metastatic liver tumors. Grants were paid for the clinical studies.